The Art of Shaping Crystals in Microgravity on Earth
- D-CHAB
- ICB
- Highlights
Growing and shaping crystals in one piece (single crystal) according to their purpose, is still hardly possible in the field of reticular solids. Dr. Josep Puigmartí-Luis (ICB) and his team showed the formation of the largest single crystals with controlled unique shapes of peptide-based metal-organic frameworks (MOFs) reported to date – produced under microgravity conditions.
Nature is an architect of stunning accuracy, as a glance at the perfectly shaped shells on the beach confirms. The precision becomes even clearer when one takes a closer look at the sand and discovers the countless elaborated shapes of the diatom shells. Here, biomineralization processes create unique, highly complex crystal structures that eventually merge to form larger bodies. The size, shape and composition of the crystals are precisely controlled, almost tailored to the function that the structures are ultimately intended to have: shells, for instance, are designed to protect – thus, the shape determines function and property.
This controlled structure is achieved by biominerals – a class of substances consisting of an organic matrix and an inorganic component. In chemistry and material science, they have long served as role models for the creation of materials. Nevertheless, their precise control over the shape and size of structures is still barely understood and unachievable in the laboratory, even though great efforts have been made to copy this system in the field of reticular solids (solids made of assembled netlike structures). However, Dr. Puigmartí-Luis (ICB, D-CHAB) and his team have now succeeded in the formation of the largest monocrystals to date with a controlled unique shape from a peptide-based organometallic framework.
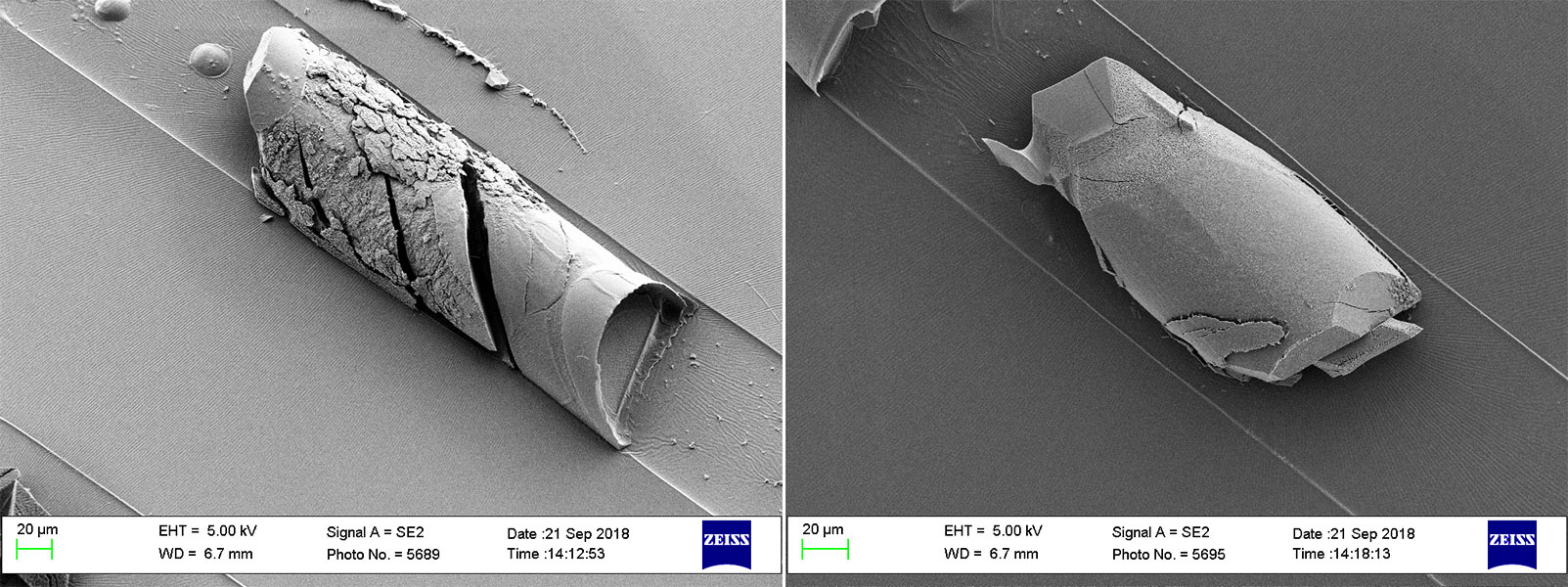
Struggling for precise control
Metal−organic frameworks (MOF) are microporous materials, where metal ions linked to organic ligands form crystalline structures, developed to produce reticular solids in a controlled manner like nature does. But there are restraints: “In the lab, you can control the shape of the pore and the properties of the ligand,” Puigmartí-Luis explains, „but the crystal growth is still uncontrolled.” This also has to do with gravity on earth and can cause problems, for instance, in the production of optical fibers. Optical fibers transmit light as well as information and are made of crystalline structures. If these crystals are not shaped precisely, light would scatter while passing through the fiber, and cannot be transmitted efficiently.
Interestingly, experiments in space showed, that microgravity leads to improved crystal growth and diffraction properties – a possible way to approximate nature’s biomineralization processes, Puigmartí-Luis thought. But working in space is expensive: “So the idea was to create microgravity mimicking conditions in the lab, where we could grow and shape crystals in a controlled manner using peptide based MOFs – a family of porous, crystalline structures that were reported recently and are capable of conformational changes, almost like proteins, thus more flexible than classical rigid MOFs..” The final product should be a unique shaped crystal grown from a solution of tripeptide glycine-L-histidine-glycine and Cu2+ (CuGHG).
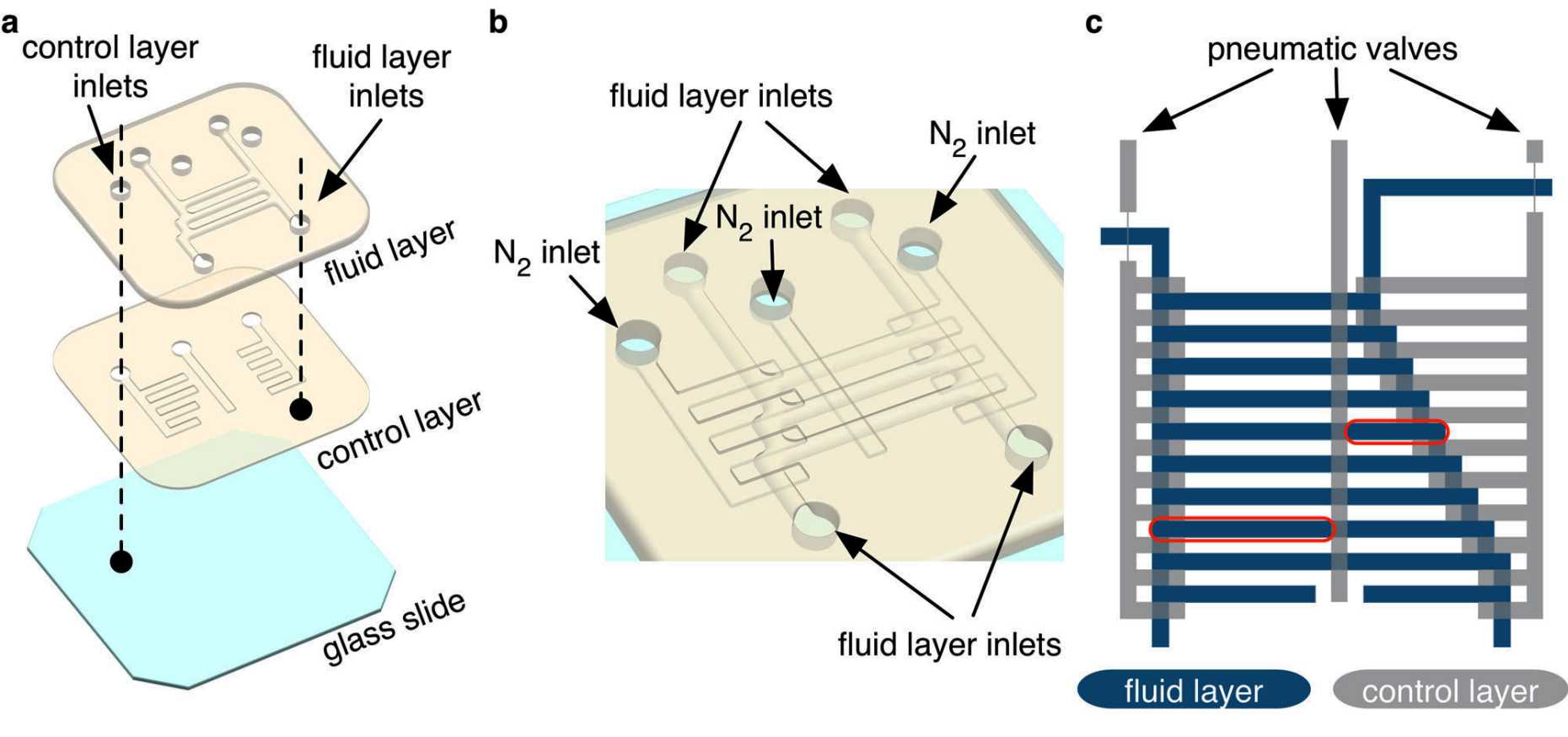
Growing crystals in microgravity
Moonlike microgravity conditions can be created on earth using microfluidic devices: microchips with channels approximately as thick as a hair, where liquids smaller than a droplet can be moved or analyzed and where viscous forces dominate. For growing and shaping CuGHG under such conditions, the group generated confined submicroliter spaces within such a microchip (Type I devices), because compartmentalization is crucial for morphogenesis in biomineralization. After the introduction of a solution of Cu2+ and peptides, the researchers could observe deep blue polyhedral CuGHG crystals growing from the precursor solution. As there are just one or two crystallizations per microchamber, they could assess also the growth kinetics at the single crystal level.
In search of extraordinary shape & size
Interestingly, looking closer at the growth process the group also observed, that “young” CuGHG crystals, thus smaller than the channel, have the shape of a polyhedron. But when they become bigger, they lose this shape and take the shape of the microchamber in which they are growing.
“If you have, for instance, peptide and copper and you do a reaction, you will always reach the most stable thermodynamic form,” Puigmartí-Luis points out, “and even the crystal shape is a thermodynamic one: the surfaces with higher energy will grow faster than others. This will give you the typical crystal form with sharp edges. If you want to generate another shape with other properties or even functions, you have to be out of this thermodynamic equilibrium. We call this type of shape out-of-equilibrium shape. That is, what we wanted to achieve with our experimental set up, and at the same time we tried to imitate the biomineralization processes of nature.”
Based on the first observations, the group aimed to prepare CuGHG crystals with more complex unique morphologies by generating Type II microfluidic devices (a branched fluid layer with nine inlets), that offer more possibilities to change parameters and monitor the effects. Within this device, the growth of a single CuGHG crystal resulted in an extraordinary branched morphology for a monolith MOF crystal – a product that would be unachievable with conventional crystallization methods according to the group.
Further experiments, again with Type I devices but without actuated pneumatic valves, led to other fascinating forms: a comb-shaped CuGHG single crystal with a size up to 2 mm in length (see header picture) and the formation of unconventional flat 2D morphologies up to 1,8 mm.
Growing, shrinking, regrowing
To gain further control over the size and shape of CuGHG single crystals, the group exposed the crystals to a constant supply of precursors under continuous flow conditions. “We chemists are used to play with things like temperature, pressure, solvent composition, or even functional moieties incorporated in building blocks to achieve what we want. But there are other things like flow that can be a key,” Puigmartí-Luis is convinced, “controlling the flow you can control chemical processes in time and space and so the outcome of your reactions, just like nature does.” Indeed, the constant supply of precursor solution turned out to be a good tool for controlling the crystal growth: for the first time, the group was able to show that above a critical concentration of about 100 mM, the crystals grow as long as they are fed and not restrained by the channel walls, otherwise they shrink.
Moreover, the microgravity-mimicking environment enabled CuGHG single crystals to cope with laser-induced damage: if the damaged zone of the crystal was still in contact with the solution, it was repaired through complete and fast regrowth, as soon as the irradiation stopped. This could be repeated up to six times.

All these findings, external page published recently in the Journal of the American Chemical Society (JACS), provide a special insight into the development of complex crystal morphologies and shed light on new methods. “We could demonstrate that using an out-of-equilibrium approach as well as microgravity mimicking conditions, and playing with fluid flows allows us to give shape and size to crystals, and this is actually what nature does.” In the future, the results will pave the way for the development of new applications for the pharmaceutical industry and material science.
external page Puigmartí et al. manuscript on the JACS Cover