Eight tons of hope: world's strongest persistent magnet for NMR at ETH
- D-CHAB
- LAC
- LPC
- Highlights
Anyone who wants to look at the atomic level to see the structure of materials, the way drugs work or viruses behave, needs great power and large equipment, in other words: several coils, umpteen kilometers of wire, all packed into potent cooling systems – in short, the world's strongest persistent magnet for nuclear magnetic resonance (NMR). It recently moved into the HCI building on air cushions and is nurturing great hopes, e.g., in virus research.
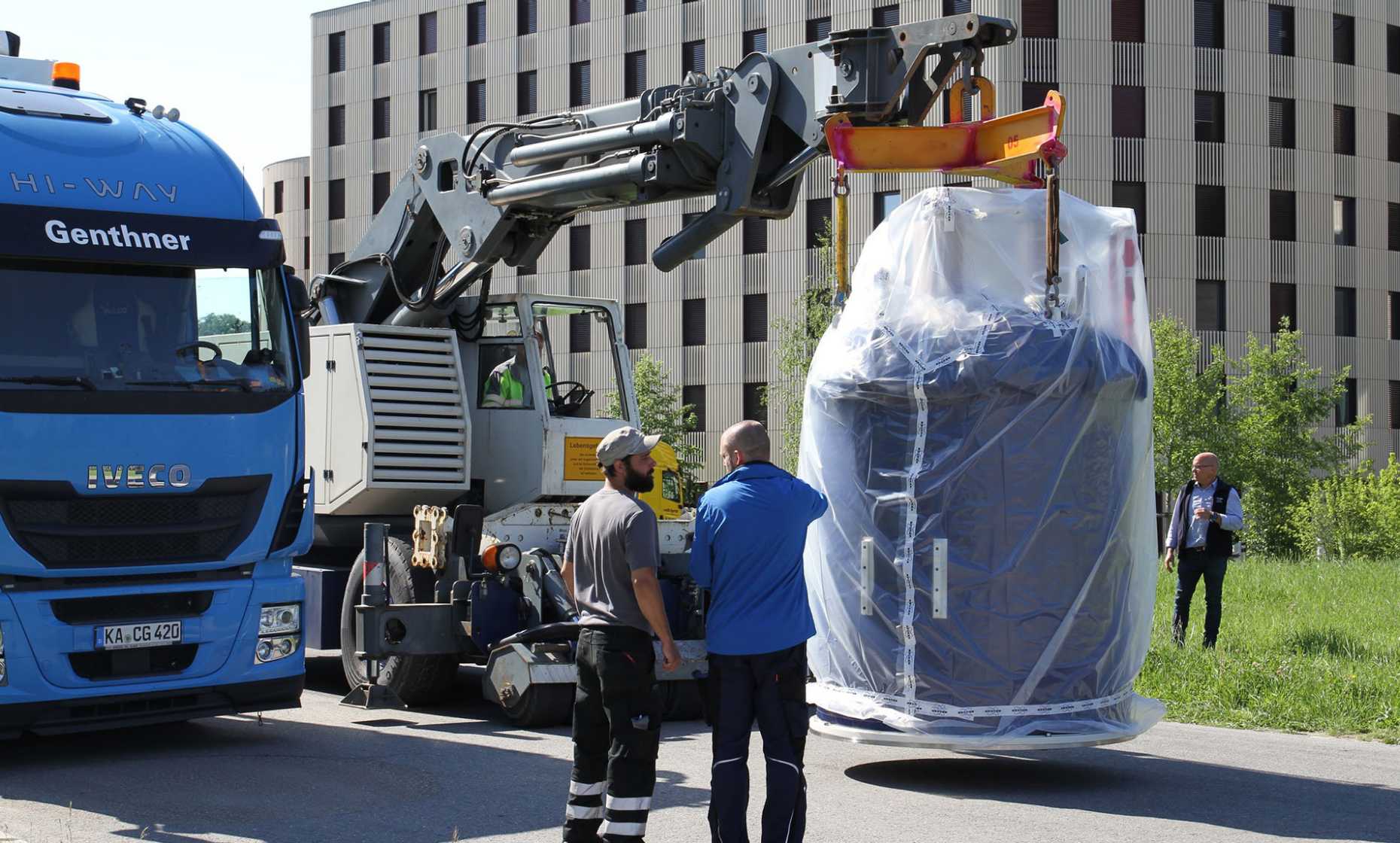
It is an exciting morning at ETH Zurich on this day in May, when a crane lifts the long-awaited, expensive freight out of the truck: a nylon-coated transport container, "ETH blue" as people enthusiastically state on Twitter, and a bit heavier than an African bull elephant. On air cushions, the world's strongest persistent magnet for nuclear magnetic resonance (NMR) with 1.2 gigahertz (GHz) and 28 Tesla (T) is moved through corridors and elevator shafts towards its destination: the hall under the 2nd pond at HCI. There, undisturbed and cooled, it will provide reliable data and new insights – especially with regard to drug effects, virus therapies and material development.
These are fantastic conditions for NMR research, Professor Beat Meier (LPC) – the moving spirit of this investment – and Professor Matthias Ernst (LPC) may have thought while observing the installation of the new magnet. As former doctoral students of Nobel Laureate Richard Ernst, they clearly remember the 1990s, when the NMR laboratory of ETH Zurich was still located near a tramline: its constantly changing magnetic field disturbed the constant field in the laboratory. "I spent half a year of my dissertation just trying to compensate for these disturbances," Matthias Ernst remembers. At that time, reliable data were only available at night – "not a very agreeable time to work", as Richard Ernst once remarked. Now, such influences can be mastered better, Ernst says. But that has been a long way.
The abstract "art" of reading molecular signals
In contrast to X-rays or cryoelectronic microscopy, NMR spectroscopy can be used to analyze molecules at room temperature and in any aggregate state. It is a method where atomic nuclei are exposed to a magnetic field and irradiated with radiofrequency pulses. After the irradiation, the nuclei "respond" with resonant frequencies that, when recorded, produce an abstract "piece of art" composed of signals. From these scientists can draw conclusions about the structure and dynamics of the molecules – as long as the signals are clear enough to be interpreted. The prerequisite for this is a strong, stable and consistent magnetic field. So the magnet is of crucial importance.
The magnetic field to which the samples / the atomic nuclei are exposed is created by coils of wire through which the electric current passes. Since the invention of NMR in the middle of the 20th century, the resonance frequency (initially around 60 MHz) and the field strength (initially around 1.4 T ≙ a strong electromagnet) have been continuously increased by optimizing the materials. Most recently, low-temperature superconducting magnets have been used: coils of wire made of materials that lose their resistance at low temperatures, thus enabling a stronger field. "Pumping on the helium, which is used to cool the materials, helps as well, because this way the boiling point of the helium drops,” Ernst explains, "nevertheless, at 1GHz and 23 T it was over. We needed a new technology.”
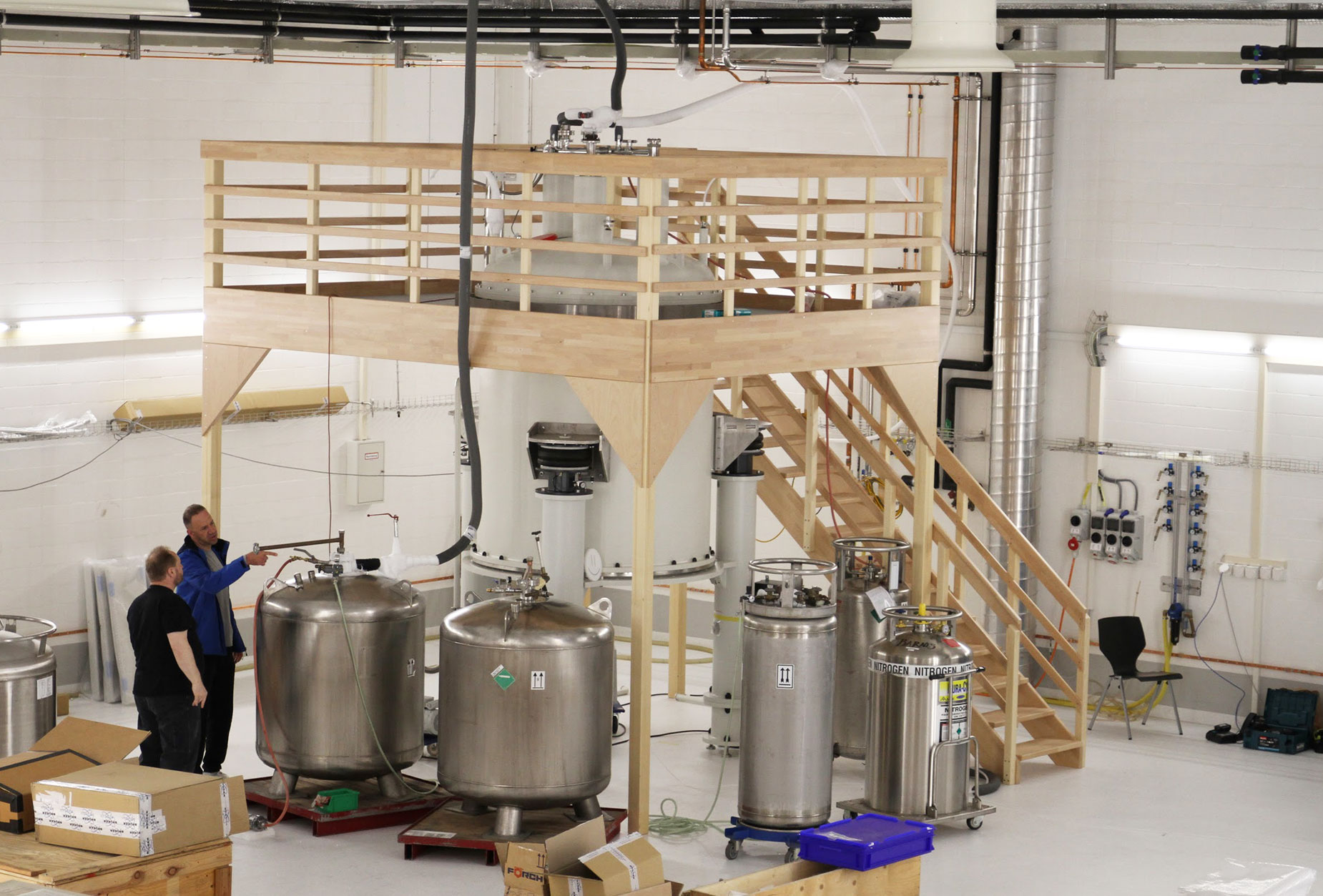
Powerful forces, deafening noise
The new persistent magnet produced by Bruker - a superconducting magnet that remains permanently magnetic without being connected to a electric power supply - works at 1.2 GHz and 28 T. That's seven times the force required for an MRI in hospital. "If something made out of iron would get into this magnet," Ernst says, "it would be impossible to remove it without turning off the magnet." This enormous force is achieved by a new combination of materials: The outer part of the magnet consists of the previously mentioned low-temperature superconductors, the inner part of high-temperature superconductors – materials that give up their resistance at higher temperatures and can withstand a higher magnetic field. Taken together, higher fields can be achieved. The magnet is cooled with liquid nitrogen and helium. It is important that the materials withstand the forces. "If not, all the energy in the magnetic field would suddenly be converted into heat," Ernst explains. That would make a deafening noise and the helium would evaporate immediately. But there are safety valves to prevent this.
Great hope: from data storage to viral therapies
The scientists have big plans now. Professor Beat Meier and his team want to use the magnet for further investigating amyloid fibrils – protein fibres that play a role in Alzheimer's disease and whose structure Meier's group was one of the first to discover. The team will also, together with french and german collaborators, focus on substances that prevent the reproduction of hepatitis viruses. "Around 200 million people have chronic, incurable hepatitis B," says Meier," NMR will help to see what happens when certain substances bind to the viral proteins. The researchers also focus on accessory proteins of the coronavirus, whose function is unclear.
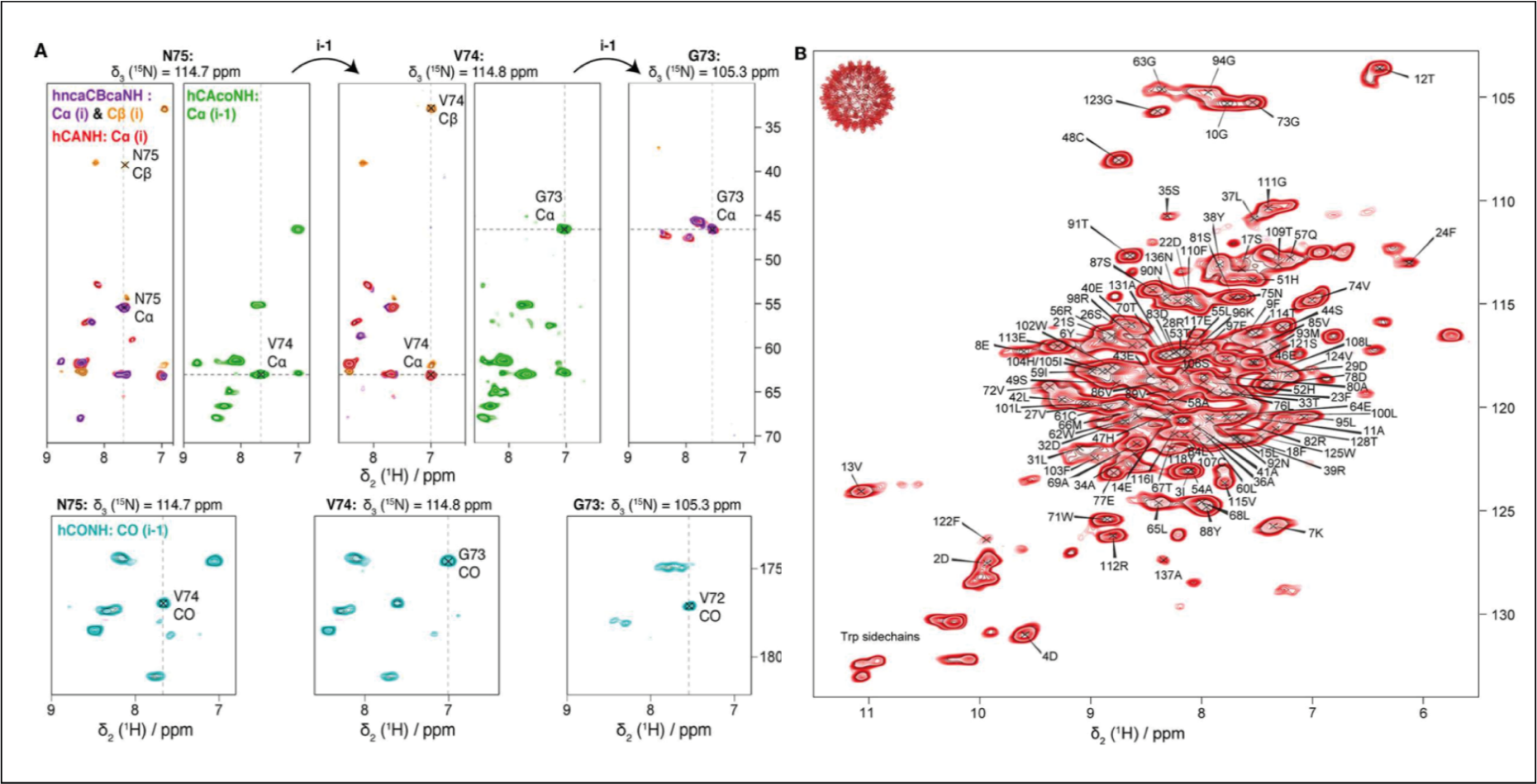
Professor Alexander Barnes (LPC) uses the magnet to further develop the NMR technology. The use of "spherical sample containers and special microwave sources will increase the performance and sensitivity of NMR by orders of magnitude," Barnes says, “we see the new magnet as a stepping stone to higher magnetic fields.” The group also has an eye towards applying this fields for in-cell structural biology. Professor Christoph Copérets group (LAC) will use NMR to investigate solid catalysts and functional materials, e.g., for energy conversion and data storage. "The new magnet makes it possible to record NMR signal with greatly improved resolution, that will enable the development of detailed structure-property relationships and ultimately the prediction of reactivity," Copéret says. The groups also plan different collaborations.
The magnet should be ready in August 2020, when it has been cooled down and charged. Apart from Zurich, only Florence in Italy has a magnet of this type. The investment will pay off, the scientists are convinced. "For top-level research, top equipment is needed," Meier says. There is great hope that this 8-tonne device "will provide completely new insights, preferably unexpected ones. These are the best."
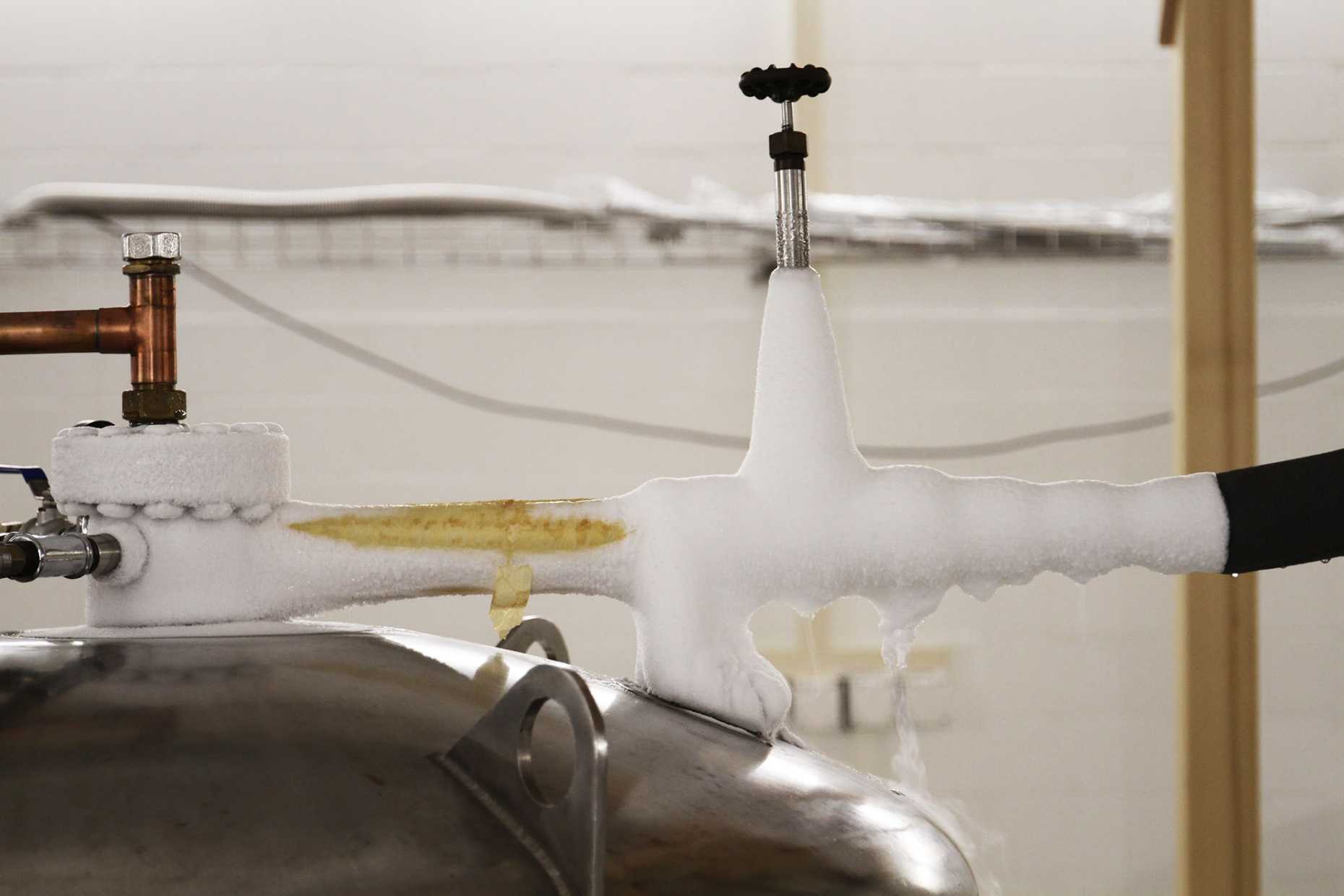
Further Information
external page Bruker 1.2 GHz NMR Magnet
Solid-State Nuclear Magnetic Resonance Group
Solid-State NMR Spectroscopy Group
Lecoq, L., Schledorn, M., Wang, S., Smith-Penzel, S., Malär, A.A., Callon, M., Nassal, M., Meier, B.H., Böckmann, A., 2019. 100 kHz MAS Proton-Detected NMR Spectroscopy of Hepatitis B Virus Capsids. Frontiers in Molecular Biosciences 6, 80–10. external page doi:10.3389/fmolb.2019.00058